Breadcrumb
- Home
- Instrumentation
Instrumentation
PIPs (Petite Ion Probes)
Instrument PI: Prof. Kristina Lynch, Dartmouth College
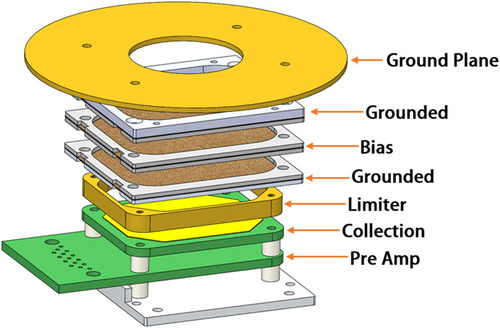
The PIPs are retarding potential analyzers (RPAs), which can be flown in situ to collect charged particles. The sensors vary a bias screen voltage in front of a collection surface and record the current on that surface as a function of charged particle flux that enters the sensor aperture and passes the bias screen; the current determines the number flux of the incoming particles, which can be either ions or electrons depending on the configuration. Multiple look directions are provided by the array of PIPs mounted around the radius of the spinning main payload. These distributed pointwise in situ observations can provide a different view of the ionosphere than is allowed by remote-sensing large-scale observations such as imaging radars.
The PIPs instruments will contribution to the LAMP objectives by measuring ion drift variability and small-scale field-aligned currents.
AIC2 (Auroral Imaging Camera 2)
Instrument PI: Associate Prof. Takeshi Sakanoi, Tohoku University
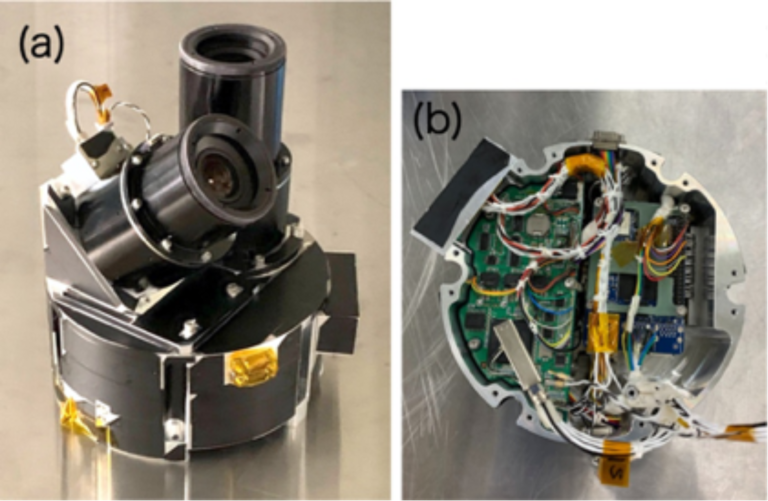
AIC2 is a multi-spectral auroral imager consists of 2 CMOS cameras, AIC-S1 and AIC-S2, and electronics AIC-E. AIC-S1 targets the auroral molecular nitrogen emission at 670 nm in the E-region with a field of view of 29 deg x 29 deg which covers 170 km x 170 km with a resolution of 3 km x 3 km at apogee. Combining the despun platform with rocket attitude control, AIC-S1 points to the magnetic footprint to carry out simultaneous observation between fine structure of pulsating aurora and precipitating electrons. AIC-S2 observes the auroral atomic oxygen emission at 844.6 nm in the F-region with a 𝜙106 deg circle field-of-view to observe the thickness of aurora and auroral distribution in the wide range. Two cameras take images simultaneously with a time resolution of 10 frame/s. The dynamic ranges of AIC-S1 and S2 are 1.1 - 860 kR, and 0.4 - 5800 kR, respectively, with a typical sensitivity resolution of a few tens Rayleighs. AIC-E consists of two NanoPi board computers, FPGAs, power supply, and signal processing electronics to handle a large amount of image data generated in two cameras. We also use a newly developed oscillating heat pipes to cool the two CPUs of NanoPi boards.
AIC2’s contribution to LAMP will be to measure the small-scale structure and the thickness of pulsating aurora with high-time and high-spatial resolutions to clarify the characteristics of pulsating aurora caused by precipitating electrons in various energy ranges.
HEP 2 (High Energy Particle detector 2)
Instrument PI: Assistant Prof. Takefumi Mitani, JAXA
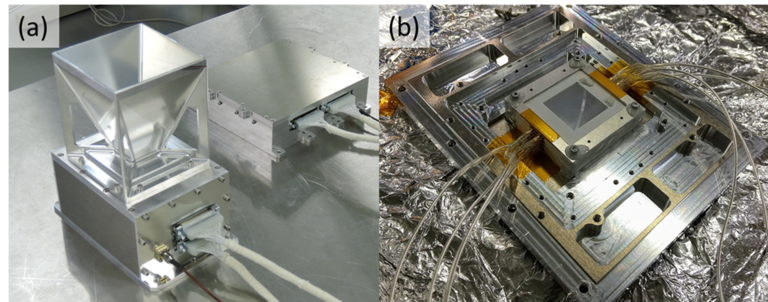
HEP2 is an improved version of High-Energy Particle detector onboard the RockSat-XN sounding rocket [Namekawa+, 2021], and designed to measure the energy spectra of electrons with energies ranging from 200 keV to 2 MeV. This sensor consists of a mechanical collimator, eight -layered silicon semiconductor detectors (SSDs) which measure the energies of incident particles, an anti-coincidence detector (a plastic scintillator and APDs) which evaluate penetrating particles such as galactic cosmic rays. The thickness of each SSD is 600 μm leading to the total thickness of all the SSDs of 4.8 mm. The field of view of HEP2 is 22.6° × 22.6° as geometrically defined by the center of the aluminum shield and the edge of the collimator. A 125 μm-thick aluminum sheet was installed just in front of the SSDs, and 18% and 70% of electrons pass through the sheet in the cases of 200 and 300 keV electrons. HEP2 can measure 975 keV electrons with energy resolution 5.5%. The output signals from two SSDs are fed together into one preamplifier to reduce the number of read-out channels, digitized by analog-to-digital converters in parallel, and sent to the ground with a time tag (24bit, 1LSB: 0.1 ms).
HEP2’s contribution to LAMP will be to measure the energy spectra of precipitating 100s keV - MeV electrons during pulsating auroras and to clarify the relationship between pulsating auroras and microbursts.
MIM (Magneto-Impedance Magnetometer)
Instrument PI: Dr. Masahito Nosé, Nagoya University
Co-I: Dr. Reiko Nomura, JAXA; Mariko Tetramoto, Kyushu Institute of Technology
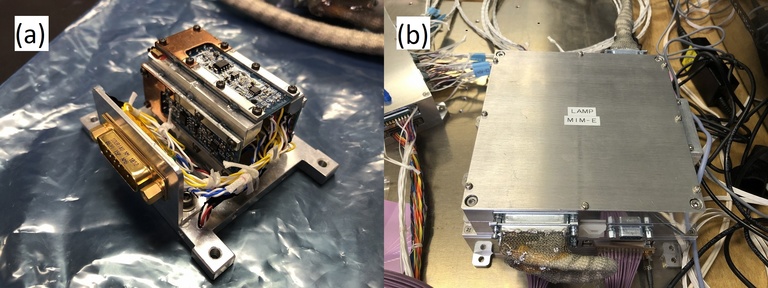
MIM is a triaxial magnetometer using “the magneto-impedance effect”, in which the impedance of an amorphous wire sensitively changes as a function of an external magnetic field when a high-frequency current is applied through the wire as a carrier. It is composed of the sensor component (MIM-S) and the data processing electronics component (MIM-E). MIM-S measures the geomagnetic field in the range of ±80,000 nT with a sampling frequency of 200 Hz and has the noise level of approximately 50 pT/√Hz. The dimensions, mass, and power consumption of MIM-S are as small as 70 mm by 70 mm by 50 mm, <0.5 kg, and ~3 W, respectively. MIM-E digitizes the output from MIM-S with a 24-bit A/D converter, resulting in a nominal resolution is ~10 pT, and processes the data with a Raspberry Pi3. Its dimensions, mass, and power consumption are 150 mm by 150 mm by 44 mm, ~1.3 kg, and ~6 W, respectively.
MIM contributes to LAMP by providing the geomagnetic field data in the ionosphere during pulsating aurora. It also makes LAMP to be the first mission that carries the magneto-impedance sensor in sounding rockets.
PLASMIC (Proton eLectron Advanced Sensor for M-I Coupling)
Instrument PI: Prof. Allison Jaynes, University of Iowa
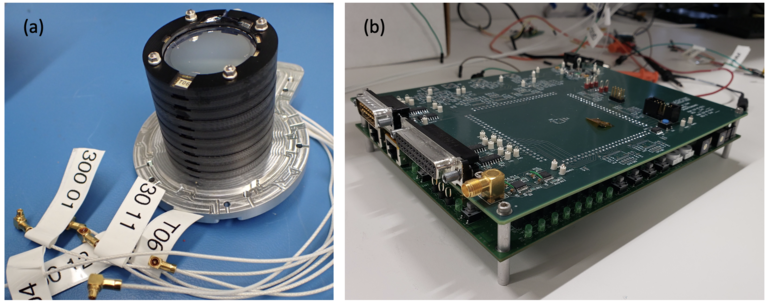
PLASMIC is a solid state detector telescope tuned for observing electrons from energies from 30 keV to 2 MeV. (It also measure higher-energy protons >1 MeV, which are not relevant for LAMP science.) PLASMIC is founded on the same principles of solid-state detector design that were featured in the highly successful REPT (onboard NASA's Van Allen Probes) and REPTile (onboard the CSSWE CubeSat) instruments. It combines a number of recent advancements in solid state detector design, modeling of energetic particle-matter interactions, digital signal processing (DSP), and the advent of capable FPGAs that can support advanced real-time characterization algorithms and counting rates up to 1x106 counts/sec. A primary benefit of utilizing DSP is pile up detection and recovery, making dead time essentially negligible after correction.
PLASMIC’s contribution to LAMP will be to measure the high energy part of the pulsating aurora spectrum to see how much of the total energy content is contained above 10s keV.